Studying these trends is an essential step in the extreme event attribution procedure we use at World Weather Attribution. Over the years, we have collected a fair number of results in these analyses and other articles. In this article, I take a step back and consider global long-term meteorological station data for hot, cold, and wet extremes, and share some thoughts on tropical cyclones and droughts. I make no claims for completeness; there is a lot of literature on this that I do not know.
The obvious first-order hypothesis is that warm extremes are getting warmer and cold extremes less cold. Severe precipitation tends to increase due to the higher moisture content of warmer air. Sea level rise simply heightens storms urges. Other extremes do not have as obvious first-order trends.
The figures are based on GHCN-D station data from NOAA/NCEI and can easily be reproduced on the KNMI Climate Explorer. They have at least 50 years of data and a minimum distance between station of 0.5º. The daily average temperature was chosen, defined as the average of maximum and minimum temperatures. This quantity is less sensitive to changes in observing practices and surroundings than either minimum or maximum temperatures alone, e.g., decreased ventilation due to trees growing affects minimum and maximum temperatures with opposite signs. There are no obvious urban/rural contrasts in the maps, so they mainly reflect large-scale trends.
Note that we compute trends as a function of the smoothed global average temperature, rather than simply time, since our first-order hypotheses are related to warming.
Heat extremes
In this analysis a heat extreme is simply defined as the highest daily average temperature of the year. Our trend analysis shows that almost everywhere these heat extremes are now warmer than a century ago, following the obvious first-order connection with global average temperature. We encountered two exceptions in our work: in the eastern U.S. heat extremes now are roughly as warm as they were during the Dust Bowl of the 1930s, when the severe drought heightened the temperature of hot days. In India, where there has generally been no trend since the 1970s, we find that increasing air pollution and irrigation counteracted the warming trend due to greenhouse gases over that period (van Oldenborgh et al, 2018). In the rest of the world, the increases are typically large, with the temperature of the hottest day of the year rising much faster than the global mean temperatures in most regions (values above one in Figure 1).
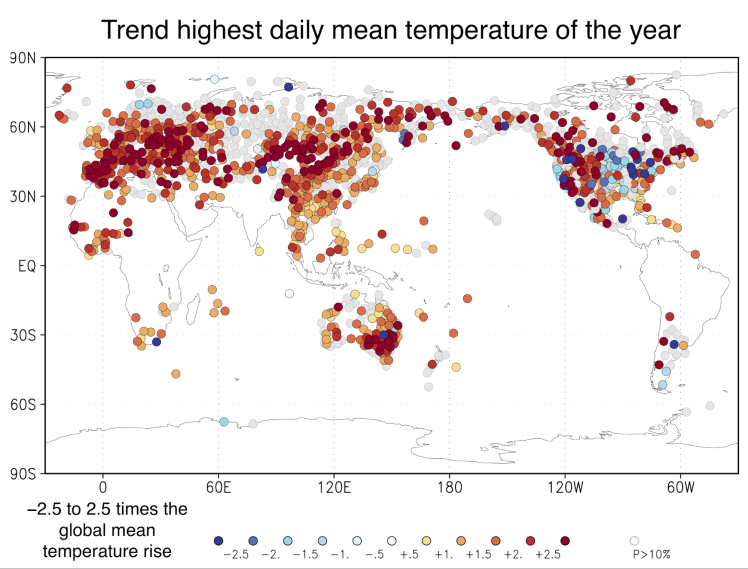
The map of Figure 1 also shows the sparsity of stations with long daily temperature records that are publicly available in the tropics. This is mainly a result of the data being sold commercially. However, in this region, temperature extremes are also often not acknowledged, although there is evidence that they pose a major health hazard (see Gerland et al, 2015 for Africa).
Cold extremes
For cold extremes, the daily average temperature of the coldest day of the year is considered. Figure 2 shows that these cold events heat up even faster than the heat extremes, up to a factor of five times the global mean temperature (see e.g., van Oldenborgh et al, 2015 and the WWA cold wave analyses). The strongest increases over land are in Siberia and Canada. Winter temperatures are very low there due to radiative cooling over snow under a clear sky, with strong vertical gradients in the lowest meters of the atmosphere. These stable boundary layers are sensitive to perturbations, probably also to the extra downward longwave radiation due to greenhouse warming. This may explain the strength of the observed trends. Further south, the cold air from the north is simply less cold, also due to the well-understood Arctic amplification over the Arctic Ocean. Note that the current climate models do not have the resolution to simulate this properly and underestimate the trends.
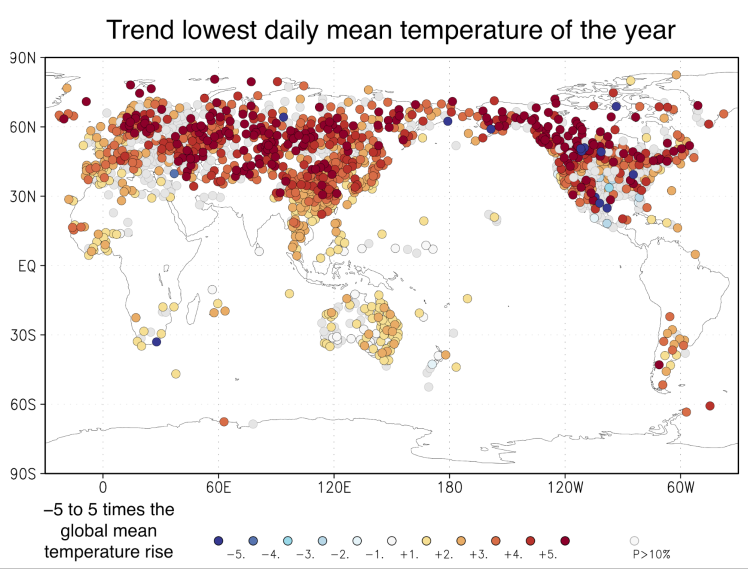
Precipitation extremes
As a measure of extreme precipitation, we take the highest daily precipitation of the year. This is a measure relevant for local flooding. For flood events over larger basins, the time over which to compute the total amount should be longer, while flash floods may be caused by shorter events, making hourly totals more relevant. The daily total is a useful measure, in the middle of the relevant temporal range. Similar maps can be made for longer times scales via the Climate Explorer. We computed trends after transforming precipitation amounts by taking their logarithm, a transformation that makes the variable more like a normal distribution so that the trend is mathematically better defined. It also ensures that the precipitation remains positive. For the trends shown here, these are almost the same as relative trends in the precipitation itself.

Note that also for precipitation, rather than computing trends in time, we compute trends as a function of global average temperature. Figure 3 shows that the highest daily mean of the year has increased at more stations than it has decreased. This was already found by Westra et al (2013). The average increase is similar to the increase of the amount of water the atmosphere can hold at higher temperatures (the Clausius–Clapeyron relation), about 7% per degree Celsius. However, there is a large spread around this average. A large part of this is random weather: even in >50-year series, the variability is large compared to the trends. In some areas, there are systematic deviations from Clausius-Clapeyron due to other effects of climate change. Some examples from my own work are listed below. The trend in Colorado is lower than Clausius-Clapeyron, probably due to higher air pressure during the season of most extremes (Eden et al, 2016). Drying trends also suppress extreme precipitation, such as in summer in the Mediterranean region. However, in autumn extremes increase strongly at one mountain range there (Vautard et al, 2015). Some winter extremes in northwestern Europe are found to increase more strongly than Clausius-Clapeyron due to the increase in zonal circulation types (e.g., van Haren et al, 2013, Schaller et al 2016), but others do not (e.g., Otto et al, 2018).
To conclude, on average daily precipitation extremes increase in intensity, but local trends are often different from the global average. We’ll be busy studying these regional trends for some time.
Tropical storms
It is hard to determine trends in the number and intensity of tropical cyclones (called hurricanes in the North Atlantic). This is because the observing system has been improved so much over the last 150 years, that more storms are detected and the most intense parts of it are more likely to be measured (Vecchi and Knutson, 2011). There is also strong decadal variability in cyclone activity in many regions. All that said, most damage of tropical cyclones is caused by water: extreme rain and storm surges. Both observations and modelling show that extreme precipitation associated with hurricanes sees large increases. For the U.S. Gulf Coast, we found an increase of about 15% in extreme precipitation, including from non-cyclone events, over the last century (van der Wiel et al, 2017; van Oldenborgh et al, 2017). Storm surges are trivially higher due to sea level rise. This means that even if the theoretically expected increase in the most intense tropical cyclones is not yet detectable, their physical impacts have increased substantially already.
Drought
Trends in drought strongly depend on the definition of drought. There are three common ones: meteorological drought, which is simply an absence of rain; agricultural drought, which is a deficit of soil moisture and thus includes evaporation (and sometimes irrigation); and hydrological drought, which also includes the transport of water. Trends in meteorological droughts are often hard to determine: drought is only a problem if the variability is large relative to the mean, but that also implies that natural variability is large compared to the trend (e.g., Philip et al, 2018, Uhe et al, 2017). Hydrological droughts, such as the one in California, can be caused by an increase in temperature rather than precipitation. This reduces the snowpack in spring and thus causes a shortage of stored water in the dry summer (e.g., Mote et al, 2016). Socio-economic drought, that is shortage of water for common use by society, is often caused by increased water use rather than decreased availability (e.g., Otto et al, 2015). It is, therefore, very hard to make general statements about drought.
Conclusions
Observations of weather extremes show the expected long-term trends in line with the increase of the global average temperature: almost everywhere hotter heat extremes, almost everywhere less frigid cold extremes, in general more intense precipitation, but with variations from region to region, and more damage from hurricanes through more precipitation and higher storm surges. Other extremes are not so simply related to climate change, and we are undertaking background research to make rapid attribution of these extremes possible.
Thanks to Claudia Tebaldi for improvements to the text. Previous versions were published as KNMI klimaatbericht and on the Climate Lab Book blog.